Engineering by nature
Learn how researchers across the College of Engineering are drawing inspiration from nature to drive cutting-edge innovations.
Researchers throughout the College of Engineering are looking to nature for inspiration to create groundbreaking innovations. Here we highlight a few recent projects where researchers take their cues from birds, dandelions, stingrays, butterflies, falling leaves and more.
David Clode / Unsplash
Stingray-inspired underwater propulsion
By studying the unique swimming abilities of stingrays and other batoids, researchers hope to unlock the secrets of efficient undulatory propulsion — ultimately informing a new generation of quiet, sustainable underwater vehicles powered by wavelike motions.
“Stingrays are fast, efficient and maneuverable swimmers that propel themselves by undulating their pectoral fins,” explains John Michael Racy, a mechanical engineering undergraduate researcher. “We want to understand the structural dynamics they use to achieve this elegant mode of propulsion.”
The research team is taking an approach that combines biological studies of batoid anatomy with physics-based modeling and experimental testing. The goal is to translate the rays’ unique structural and dynamic characteristics into practical engineering designs.
Racy notes the diversity in body shapes, skeletal structures and swimming modes among batoid species, which can provide insights for bio-inspired robotic designs adapted to different scenarios. Current underwater vehicle designs use complicated systems to create motion, but batoids are able to achieve similar motion more simply by recycling ocean wave energy through a network of specialized musculoskeletal elements.
In particular, the team thinks that batoids have developed special fins that move on their own. These fins have different stiffness in different parts, which helps them make waves when they move. Experimental and finite element models have demonstrated this effect, suggesting that this approach could simplify actuation and control for robotic undulatory propulsion.
Ed Habtour, an assistant professor of aeronautics and astronautics, emphasizes the project's interdisciplinary nature, combining biology, dynamics and engineering: “By uncovering the core mechanisms behind batoids’ extraordinary swimming talents, [the team’s] insights could catalyze transformation in underwater vehicle design.”
Boris Smokrovic / Unsplash
Exploring the world through butterfly eyes
Most people notice the beauty of butterfly wings. But for Gary Bernard, an electrical and computer engineering affiliate professor and alumnus (BSEE ‘59, MSEE ‘60, Ph.D. ‘64), butterfly eyes are what he finds most captivating. Investigating their vision has, in fact, become his life’s work.
At his home laboratory, UW ECE affiliate professor and alumnus Gary Bernard reflects on his prolific career researching butterflies. Photo credit: Ryan Hoover
“How does a small butterfly eye behave so beautifully and do such amazing things?” asks Bernard, who has held positions at MIT, Yale and The Boeing Company. “The technological fallout from our work has been absolutely amazing.”
It all started in 1966, when Bernard made a notable scientific discovery after placing a butterfly under a microscope. Through the lens, he saw a green reflection from the butterfly eye, which quickly disappeared. The next time he looked, it mysteriously changed to red. Now known as butterfly eyeshine, this phenomenon is caused by a reflector behind the retina. Since it is most often found in nocturnal animals, it’s still a mystery why eyeshine occurs in butterflies — which are active only during the day.
The discovery of butterfly eyeshine set Bernard on the research path he still follows to this day, investigating how light can be used as an optical probe to learn about butterfly vision. Since then, he and his colleagues have also discovered that some species of butterflies have high visual acuity due to the ability to see polarized light. The research has had notable impact, including advancing machine vision and real-time monitoring of manufacturing processes.
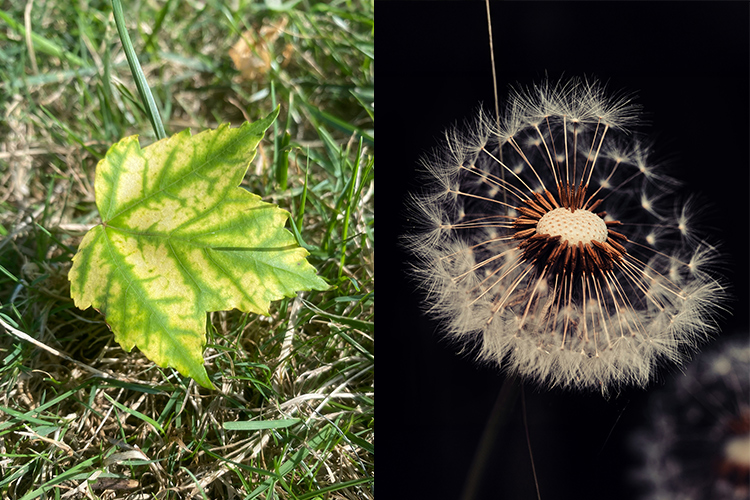
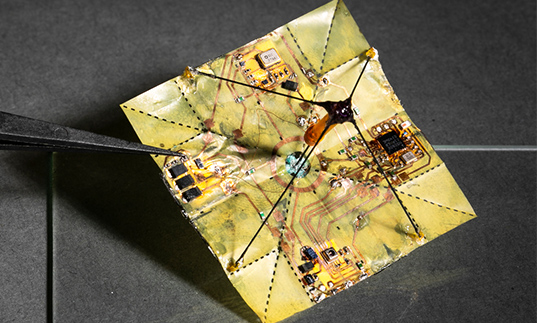
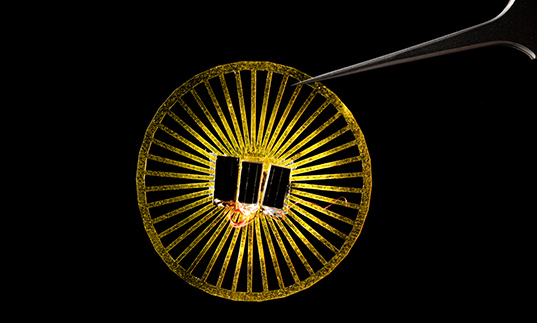
Left: Star of the Sea / Unsplash. Middle: Tobias Kaiser / Unsplash. Top right: Taking inspiration from an origami design informed by leaves, UW researchers developed a small robotic device called a "microflier" that snaps into a folded position during its descent. Mark Stone/University of Washington. Bottom right: Modeled after how dandelions use the wind to distribute their seeds, UW researchers designed a tiny sensor-carrying device. Mark Stone/University of Washington
The nature of falling
Taking inspiration from dandelion seeds and leaves, Vikram Iyer, an assistant professor in the Paul G. Allen School of Computer Science & Engineering, has two projects that advance the dissemination of sensing devices and miniature wireless robots.
For the first project, Iyer’s team developed a tiny sensor-carrying device that is modeled after how dandelions use the wind to distribute their seeds. This device can travel up to 100 meters in a moderate breeze, which provides a way to quickly distribute hundreds of tiny wireless sensors that could monitor conditions across large plots of land, from forests to farms.
“The way dandelion seed structures work is that they have a central point and these little bristles sticking out to slow down their fall. We took a 2D projection of that to create the base design for our structures,” explains Iyer. “We added a ring structure to make it more stiff and take up more area to help slow it down.”
Iyer’s lab also took inspiration from a geometric pattern found in leaves, which informs the Miura-ori origami fold, to develop small robotic devices called “microfliers.” They snap into a folded origami position during their descent, which allows researchers to control where they land.
“In its unfolded flat state, our origami structure tumbles chaotically in the wind, similar to an elm leaf,” says Iyer. “But switching to the folded state changes the airflow around it and enables a stable descent, similarly to how a maple leaf falls.”
Rhys Kentish / Unsplash
How bird behavior can advance autonomous vehicles
When the light turns green in an intersection, there’s a delay between when the driver in the first car advances and when the driver in the eighth car advances. Even autonomous vehicles aren’t able to start moving at the same time. More vehicles would be able to cross the intersection at the same light if they all “knew” the light was turning green, started together and continued moving together.
Traffic is one area studied by Santosh Devasia, a professor of mechanical engineering, who recently improved a mathematical model describing how birds flock together while suppressing unwanted noise. This algorithm could be applied to build robots that work together better — such as a fleet of self-driving cars, or robots working in a large group to carry an item without damaging it.
In swarms of birds, information propagates without distortion. For example, to avoid predators, a flock of starlings can perform parallel sharp turns. Devasia’s model takes into account that each bird adjusts its actions based on observations from its neighbors as well as its own previous actions, a concept he calls delayed self-reinforcement. His improved model shows that delayed self-reinforcement can reduce distortion during information propagation, even in noisy environments. This model could be used to improve cohesion in engineered networks, such as autonomous drone formations and in traffic.
“Our method removes the high-frequency noise,” Devasia says. “You don’t want to follow the noise; you want to follow the motion.”
Related story
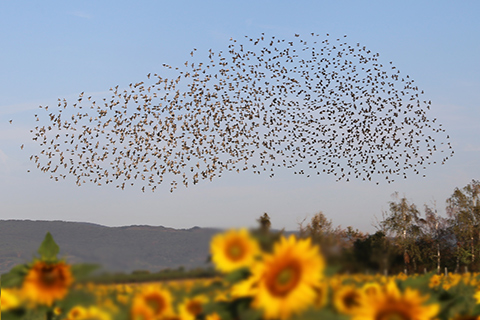
The murmuration whisperer
Santosh Devasia investigates how birds’ flocking behavior can inform autonomous robotics research.
Engineering excellence for the public good
Learn more about how we are creating a healthier and more just world through our work.